Single-Molecule Magnets (SMMs)
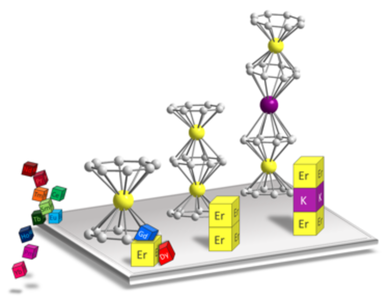
The digital economy currently represents 10% of the world’s electricity consumption, and its energy demands continue to increase. Single-Molecule Magnets (SMMs) offer a novel solution to curbing those demands, by processing and storing information at the molecular level to produce more high-performance energy-efficient electronics: with higher computing speeds and high-density data storage. Measuring one-billionth of a metre, they are poised to revolutionize energy-saving electronics and quantum computing. SMM-based hard drives will be able to store 30 terabits of data per square centimetre – hundreds of times more than the current best commercial hard drives – while using significantly less energy.
The chief hurdle is that single-molecule magnets only operate at extremely low temperatures. To be practical, they must be able to perform at room temperature while retaining their magnetic memory, without the use of expensive coolants. The Murugesu research group is working on developing high-performing SMMs based on lanthanide ions, elements that have great optical and magnetic properties, to create the next generation of magnetic materials for molecular electronics.
Join us in this exciting challenge.
The chief hurdle is that single-molecule magnets only operate at extremely low temperatures. To be practical, they must be able to perform at room temperature while retaining their magnetic memory, without the use of expensive coolants. The Murugesu research group is working on developing high-performing SMMs based on lanthanide ions, elements that have great optical and magnetic properties, to create the next generation of magnetic materials for molecular electronics.
Join us in this exciting challenge.
Opto-Magnetic Materials
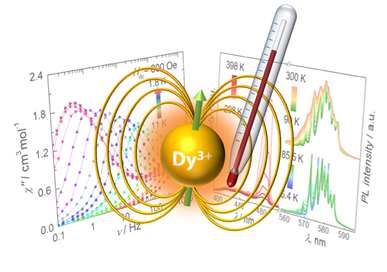
Lanthanide ions are ideal candidates for the preparation of high-performance SMMs, but their potential does not end there. Their electronic structure endows them with unique optical properties, making lanthanide ions a prime choice for the preparation of luminescent materials. Applications of such materials encompass phosphors for anti-counterfeiting and lighting purposes, and luminescent probes for application in ion, (bio)molecule and temperature sensing, to name a few.
In the Murugesu Group, we aim to harness the full potential of materials based on lanthanide ions creating molecular systems that combine magnetic behaviour and photoluminescence.
Pivotal to the realization of such materials, is the rational design of organic ligands capable of simultaneously ensuring magnetic anisotropy and sensitizing the lanthanide luminescence – which is otherwise intrinsically dim. From a fundamental standpoint, valuable information can be retrieved from the luminescence signal in order to interpret the processes involved in the magnetic relaxation. On a more practical note, the amalgamation of these features into a single entity opens thrilling possibilities for the preparation of multifunctional materials operating at the molecular scale.
Indeed, a major focus of our research is the preparation of (what we envision to be) building blocks for ultra-miniaturized, magneto-electronic devices where addressability of each component is granted by their stimuli-dependent optical properties. The quest for colourful, multipurpose materials has only just begun!
In the Murugesu Group, we aim to harness the full potential of materials based on lanthanide ions creating molecular systems that combine magnetic behaviour and photoluminescence.
Pivotal to the realization of such materials, is the rational design of organic ligands capable of simultaneously ensuring magnetic anisotropy and sensitizing the lanthanide luminescence – which is otherwise intrinsically dim. From a fundamental standpoint, valuable information can be retrieved from the luminescence signal in order to interpret the processes involved in the magnetic relaxation. On a more practical note, the amalgamation of these features into a single entity opens thrilling possibilities for the preparation of multifunctional materials operating at the molecular scale.
Indeed, a major focus of our research is the preparation of (what we envision to be) building blocks for ultra-miniaturized, magneto-electronic devices where addressability of each component is granted by their stimuli-dependent optical properties. The quest for colourful, multipurpose materials has only just begun!
Energetic Materials
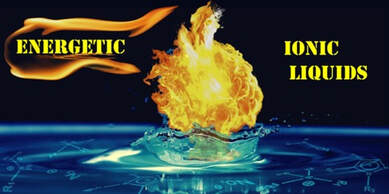
The drive to discover new energetic materials that can enhance different explosive parameters – such as detonation pressure or velocity – has led to increased activity within this field of research. That being said, many of the often-used compounds in industry consist of heavy-metal derivatives, which typically contain lead or mercury, rendering many industrial explosives as environmentally problematic.
The development of metal-free, nitrogen-rich energetic materials however, is attractive not only for their large positive standard enthalpies and high thermal stabilities, but also because their decomposition products are environmentally benign (primarily consisting of N2 gas). This has many important industrial implications, from usage in mining operations to new generations of rocket propellants.
By using various nitrogen-rich organic moieties such as pyrazines, tetrazines, and tetrazoles, previously unexplored energetic materials can be obtained. These compounds can be further extended into families of either cationic or anionic salts, which can not only enhance the energetic properties of the material but may even affect a change from a secondary explosive (e.g. stable material requiring an initiator) to a primary explosive (e.g. highly sensitive materials used to ignite secondary explosives).
Our group has interests in developing green energetic materials with excellent detonation parameters, and would welcome you to help us push the frontiers of this research forward.
The development of metal-free, nitrogen-rich energetic materials however, is attractive not only for their large positive standard enthalpies and high thermal stabilities, but also because their decomposition products are environmentally benign (primarily consisting of N2 gas). This has many important industrial implications, from usage in mining operations to new generations of rocket propellants.
By using various nitrogen-rich organic moieties such as pyrazines, tetrazines, and tetrazoles, previously unexplored energetic materials can be obtained. These compounds can be further extended into families of either cationic or anionic salts, which can not only enhance the energetic properties of the material but may even affect a change from a secondary explosive (e.g. stable material requiring an initiator) to a primary explosive (e.g. highly sensitive materials used to ignite secondary explosives).
Our group has interests in developing green energetic materials with excellent detonation parameters, and would welcome you to help us push the frontiers of this research forward.
Nanoporous Materials

Nanoporous materials called Metal-organic Frameworks (MOFs) are leading candidates in the replacement of older technologies in dealing with gas storage, catalysis and molecular separation.
We have a particular focus on MOFs that behave as crystalline sponges for the study of dynamic adsorption behaviours. In order to achieve this, we take advantage of the inherent crystallinity and rigidity of MOFs, to study the inclusion behaviours in a single-crystal-to-single-crystal fashion. This allows for unprecedented insights into unique and dynamic sets of binding motifs.
We are also currently investigating the potential of MOFs to remove CO2 from flue gas in fossil fuel-based power plants. We are performing this research in conjunction with computational resources that assist us in identifying key functional groups and pore topologies, that would lead to high CO2/H2 selectivities and uptake capacities. Finally, we have a strong interest in the rational design of cationic frameworks that enable anion-exchange processes for the efficient capture of toxic anions. While a plethora of synthetic methodologies exist for tuning nearly every aspect of neutral MOFs, we seek the controlled generation of cationic frameworks without inducing significant chemical changes to the original material.
Join us in the pursuit of the next generation of MOFs.
We have a particular focus on MOFs that behave as crystalline sponges for the study of dynamic adsorption behaviours. In order to achieve this, we take advantage of the inherent crystallinity and rigidity of MOFs, to study the inclusion behaviours in a single-crystal-to-single-crystal fashion. This allows for unprecedented insights into unique and dynamic sets of binding motifs.
We are also currently investigating the potential of MOFs to remove CO2 from flue gas in fossil fuel-based power plants. We are performing this research in conjunction with computational resources that assist us in identifying key functional groups and pore topologies, that would lead to high CO2/H2 selectivities and uptake capacities. Finally, we have a strong interest in the rational design of cationic frameworks that enable anion-exchange processes for the efficient capture of toxic anions. While a plethora of synthetic methodologies exist for tuning nearly every aspect of neutral MOFs, we seek the controlled generation of cationic frameworks without inducing significant chemical changes to the original material.
Join us in the pursuit of the next generation of MOFs.
2008-2022 © The Murugesu Group
Department of Chemistry and Biomolecular Sciences | Faculty of Science | University of Ottawa
Department of Chemistry and Biomolecular Sciences | Faculty of Science | University of Ottawa